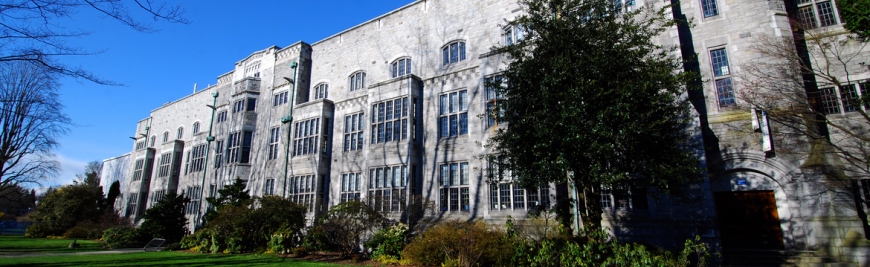
Ido Levin
Profile
Research and Teaching Interests
We take inspiration from nature to design and fabricate programmable and active materials. Unlike traditional materials, the actuation of our materials is distributed continuously, allowing for better adaptability and flexibility. We're pioneering new approaches combining geometrical modeling with novel materials and innovative fabrication, paving the way for the next generation of soft machines.
Programmable responsive materials
The discovery of responsive materials, together with advancements in fabrication techniques, resulted in a great interest in programmable materials - materials that can be encoded with a desired shape, actuable using external stimuli. Our research combines innovative fabrication approaches with diverse responsive materials, often integrating novel materials developed by our research partners. We complement our experimental work with cutting-edge modeling to understand and optimize shape-transformation mechanisms.
A key focus of our work is developing materials that can respond to multiple different stimuli. This multi-responsiveness enables us to encode several distinct shapes within a single material, each activated by its own specific trigger. By activating these triggers simultaneously, we can achieve an extensive range of shape configurations.
Beyond shape transformation, we're exploring how programmable materials can exhibit unique mechanical behaviors, particularly in terms of flexibility and deformation. We're developing rigorous methods to both design these mechanical properties and accurately measure them in our engineered materials.
Chemo-mechanical active solids
While traditional programmable materials rely on external triggers for actuation, nature shows us a different path. Living tissues, like the heart, transform their shape through internal mechanisms - electrical signals propagate through the tissue, triggering localized contractions that create coordinated shape transformations. We're replicating this elegant process using specialized gels that incorporate autocatalytic chemical reactions that induce local contractions that are translated to a global change of shape. These self-oscillating gels harvest chemicals from their environment to fuel the reaction, resulting in an autonomous shape transformation.
Our current research aims to create materials with distinct functional zones - active regions that serve as artificial muscles, working alongside static supporting sections. This targeted approach to material activity allows us to engineer more sophisticated and efficient shape-changing systems. We are working to realize these materials through 3D printing and fabricating double-network gels.
Patterning material properties of synthetic lipid membranes via phase separation
We create artificial membranes that mimic cell membranes' ability to self-organize through phase separation, where different lipid components naturally sort themselves into distinct regions. This organization process, which cells use to create functional structures, significantly influences membrane mechanics. Understanding and controlling this behavior could enhance the performance of synthetic membranes, particularly for applications like drug delivery.
However, the patterns that typically emerge during phase separation are relatively simple. Our research focuses on developing methods to generate and manipulate more sophisticated domain patterns while studying how these patterns interact with membrane shape and mechanical properties. This work serves dual purposes: advancing the capabilities of engineered membranes while deepening our understanding of how phase separation functions in biological systems.
Geometric foundations of shape morphing in soft systems
We combine analytical and numerical tools to study a variety of problems regarding the geometry and mechanics of soft-matter systems. These are usually motivated and accompanied by experimental work conducted in our lab or by some of our collaborators, translating physical observations into mathematical frameworks. Examples are shaping mechanisms and shape transitions in growing thin sheets and self-assembled macromolecules, mechanical instabilities, transport phenomena and pattern formation on curved and evolving surfaces, and fluid-structure interactions.
Our approach is usually geometric, expressing energetics as functions of the intrinsic and extrinsic geometries of the structure. We are particularly interested in “frustrated” systems, systems that are residually stressed due to competing constraints. These systems reveal rich physical behaviors, including spontaneous symmetry breaking and the emergence of complex patterns.
Contact
Curriculum Vitae
2025- present: Assistant Professor, Departments of Chemistry and Mathematics, University of British Columbia, Vancouver (starting August 2025)
2022-2025: WRF Postdoctoral Fellow, Department of Chemistry, University of Washington, Seattle
2021-2022: Fulbright Postdoctoral scholar, Department of Chemistry, University of Washington, Seattle
2015-2021: Department of Physics, Hebrew University of Jerusalem, Israel (Ph.D., 2021)
2012-2014: Department of Physics, Hebrew University of Jerusalem, Israel (M.Sc., 2021)
2009-2012: Departments of Physics and Mathematics, Hebrew University of Jerusalem, Israel (B.Sc., 2021)